Superconductivity is a
phenomenon observed in several metals and ceramic materials.
When these materials are cooled to temperatures ranging from
near absolute zero ( 0 degrees Kelvin,
-273 degrees Celsius) to liquid nitrogen temperatures (
77 K, -196 C), their electrical resistance drops with a
jump down to zero.
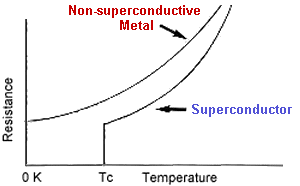
The
temperature at which electrical resistance is zero is called the
critical temperature (Tc)
and this temperature is a characteristic of the material as it
is shown in the following table:
Material |
Type |
Tc(K) |
Zinc |
metal |
0.88 |
Aluminum |
metal |
1.19 |
Tin |
metal |
3.72 |
Mercury |
metal |
4.15 |
YBa2Cu3O7 |
ceramic |
90 |
TlBaCaCuO
|
ceramic |
125 |
The value of the critical temperature is dependent on the
current density and the magnetic field as shown in this
picture.
The cooling of the materials is achieved using liquid
nitrogen or liquid helium for even lower temperatures.There
is already in this small table a clear separation between the
low and high temperature superconductors. While
superconductivity at low temperature is well understood, there
is no clear explanation as yet of this phenomena at "high
temperatures". The
critical temperature is known to be inversely proportional to
the square root of the atomic mass. Take a look at the periodic table
, to see which elements have been found to
have superconducting properties.
Some background .....
Electrical resistance in metals arises because electrons
moving through the metal are scattered due to deviations from
translational symmetry. These are produced either by
impurities, giving raise to a temperature independent
contribution to the resistance, or by the vibrations of the
lattice in the metal.
In a superconductor below its critical temperature, there is
no resistance because these scattering mechanisms are unable to
impede the motion of the current carriers. As a negatively-charged electron
moves through the space
between two rows of positively-charged atoms, it pulls inward on the
atoms of the lattice. This distortion
attracts a second electron to move in behind it.
An electron in the lattice can
interact with another electron by exchanging an acoustic quanta
called phonon. Phonons
in acoustics are analogous to photons in electromagnetic. The
energy of a phonon is usually less than 0.1 eV (electron-volt)
and thus is one or two orders of magnitude less than that
of a photon.
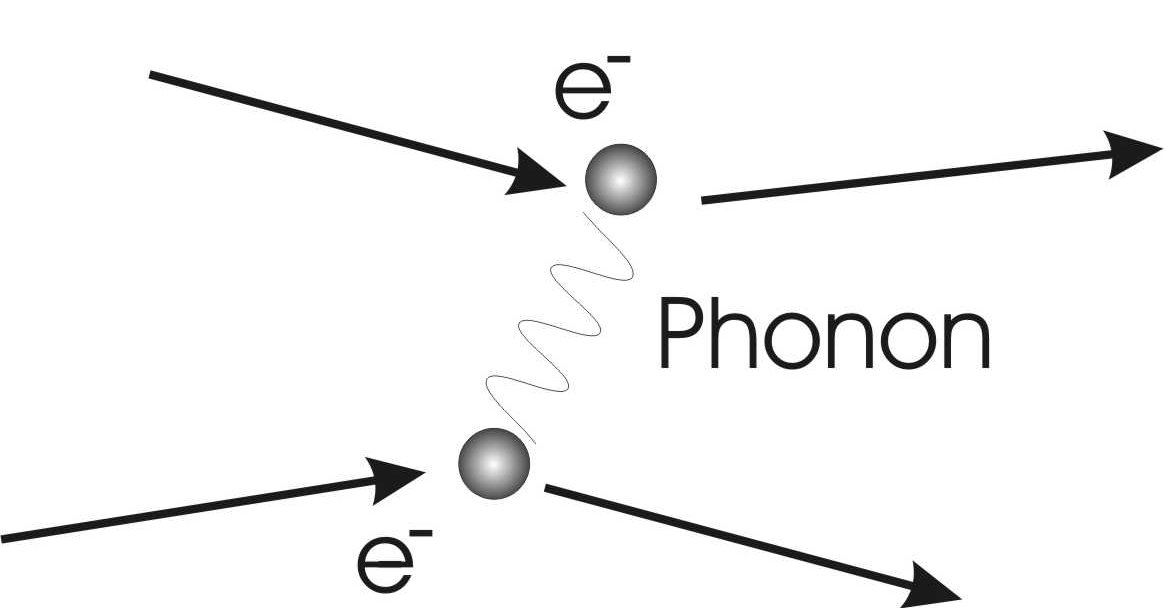
The two electrons form a weak attraction, travel together in a
pair and encounter less resistance overall. In a superconductor,
electron pairs are constantly forming, breaking and reforming,
but the overall effect is that electrons flow with little or no
resistance. The current is
carried then by electrons moving in pairs called Cooper
pairs.
A Cooper Pair moving through the lattice
The second electron encounters less resistance, much like a
passenger car following a truck on the motorway encounters less
air resistance.
Below the critical temperature these superconducting
materials have no electrical resistance and so they can carry
large amounts of electrical current for long periods of time
without loosing energy as ohmic heat. For example, loops of
superconducting wire
have been shown to carry electrical currents for several years
with no measurable loss. This property offers tremendous
challenges and opportunities in the modern world.
MEISSNER EFFECT
Another property of superconducting materials is the Meissner
Effect. It was observed that as a magnet is brought near a
superconductor, the magnet encounters a repulsive force.
It can be said that the superconductor completely expels the
magnetic field and behaves as a perfect diamagnet.
The classic demonstration of the Meissner
Effect.
A superconductive disk on the bottom, cooled by liquid
nitrogen, causes the magnet above to levitate. The floating
magnet induces a current, and therefore a magnetic field, in the
superconductor, and the two magnetic fields repel to levitate
the magnet.
This property has implications for
making high speed, magnetically-levitated trains, for making powerful, small, superconducting magnets
for magnetic resonance imaging, etc. JOSEPHSON EFFECT One other property of superconductors is that when two of
them are joined by a thin, insulating layer, it is easier for
the electron pairs to pass from one superconductor to another
without resistance . This is called the Josephson Effect. This effect has
implications for superfast electrical switches that can be used
to make small, high-speed computers. SPECIFIC HEAT In
a superconducting phase transition, the electric resistance
changes with a jump, while the energy undergoes a continuous
variation. The specific heat, or the amount of heat
necessary to affect its temperature, also changes with a
jump. When a substance is cooled, its specific heat
typically decreases but at the critical temperature it increases
suddenly. SUPERFLUIDITY This
phenomenon was first observed in helium at a temperature below
2.17K. Helium at these low temperatures was seen to flow
quite freely, without any friction, through any gaps and even
through very thin capillary tubes. Once
set in circular motion, for example, it will keep on flowing
forever - if there are no external forces acting upon it. Unlike
all other chemical elements helium does not solidify when cooled
down near absolute zero. Physicists explain this phenomenon by
extremely weak attractive forces between the almost
"perfectly round" atoms and by their rapid motion
which is due to Heisenberg's Uncertainty Principle
Bulk superfluid helium has many unusual properties - it can flow
up walls and through narrow pores without resistance. Helium-4
and Helium-3 become superfluid below 2.12 and 0.003 Kelvin
respectively. However, only a proportion of the Helium becomes
superfluid at the transition temperature.
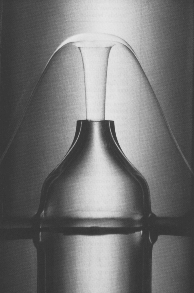 This
free movement of helium at a temperature below 2.17K looks very
much like the superconductivity behaviour mentioned
above. To explain this frictionless motion, we can
imagine that all the particles in the liquid are linked together
and none of them can be separated, without violating the whole
state. CONCLUSION Both
these two special properties were described by Ziman with a very
apt epigram: "The more of us gather, the
merrier we are together".
The future of superconductivity research is to find materials
that can become superconductors at room temperature. Once this
happens, the whole world of electronics, power and
transportation will be revolutionized.
For more information,
try these links:
HowStuffWorks
look there for "superconductivity" . You can even find
there questions for students
Making High-Temperature Superconductors
Phonon Java program
shows the influence of the lattice to the moving electron
Superconductor Modelling, GJ Barnes
Superconductors
with many LINKS
WONDERMAGNET.COM - Wholesale Neodymium and ceramic magnets, superconductors, and other fascinating stuff
Superconducting technology
at the Homepage of LHC - Project at CERN
|